Nitrogen, the seventh element of the periodic table, is a rather underrated but critical component to the functioning of all life on Earth. The colorless, odorless, and tasteless di-nitrogen (N2) gas constitutes 78 percent of Earth’s atmosphere. Besides its prevalence in the air we breathe, nitrogen is essential to the building blocks of life, namely, our DNA, RNA, and proteins. Like most things on Earth, this element is cycled through the atmosphere, biosphere, and geosphere, undergoing necessary chemical and biological transformations in order to be used to its fullest capacity. From air to soil, to plants and animals, this biogeochemical cycle feeds nitrogen to organisms that absorb and assimilate, then return back to the atmosphere and soil, fueling life as we know it.1
2
There are four major processes that contribute to the nitrogen cycle: nitrogen fixation, nitrification, decay (or ammonification), and denitrification.3 Let’s take a closer look at these 4 processes.
Nitrogen Fixation
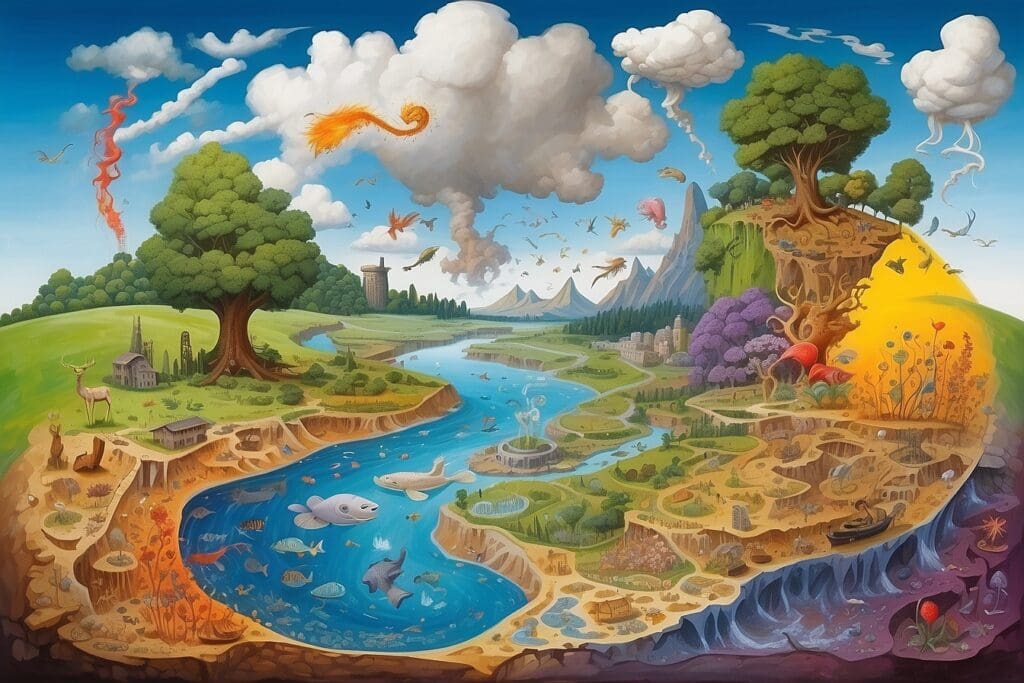
Nitrogen fixation is the means by which atmospheric nitrogen is broken down into two lone nitrogen atoms. This allows the nitrogen to combine with either hydrogen or oxygen to form compounds such as ammonia and nitrogen oxides, respectively.4 The fixation process is particularly noteworthy, as it is the only way nitrogen gas can be made productive for use by organisms. Nitrogen fixation can be achieved through atmospheric fixation (via lightning), biological fixation (via relationships with microbes), and industrial fixation (via scientific processes involving a great deal of pressure, temperature, and energy), discussed further below:
- Atmospheric fixation: This particular process accounts for approximately 5 – 8 percent of all fixed nitrogen.5 During atmospheric fixation, the tremendous force of lighting is powerful enough to dissociate the di-nitrogen triple bond, freeing nitrogen atoms and allowing them to combine with oxygen in the atmosphere to form nitrogen oxides. These molecules will then dissolve in the rain to form nitrates (NO3-), which are deposited to the Earth and incorporated into the biosphere.6
- Biological fixation: Biological fixation typically results from a symbiotic relationship between nitrogen-fixing bacteria and a host plant, most commonly in the legume family, such as beans, peas, and alfalfa.7 In fact, twenty percent of biologically fixed nitrogen is attributed to this kind of symbiosis, in which bacteria (Rhizobium) inhabit small root nodules of its host plant. The bacteria exist in an altered form called bacteriodes, providing accessible forms of nitrogen to its host in exchange for carbohydrates. Aside from Rhizobium are free-living bacteria, such as Azotobacter, that can fix nitrogen without the assistance of a host plant, as well as bacteria that establish mutual relationships with animals, such as termites.8 Much like atmospheric fixation, the bacteria are able to break apart molecular nitrogen, enabling the nitrogen atoms to combine with hydrogen to form ammonia (NH3), which is then readily available in the soil for absorption and assimilation by plants.
- Industrial Fixation: Founded by two German Chemists, Fritz Haber and Carl Bosch, the Haber-Bosch process is renown for its ability to produce synthetic ammonia, which is a major component of most commercial fertilizers. The invention has since transformed the agriculture industry and global food system, for better or for worse, and was seen as particularly auspicious during the First World War when the use of fertilizer drastically increased food production for war-ridden countries.9 The Haber-Bosch process, or industrial fixation, combines atmospheric nitrogen with hydrogen at a temperature of 600ºC to form ammonia.10 While ammonia can be used as fertilizer, it is typically converted into urea or ammonium nitrate.
https://web.archive.org/web/20160404103032if_/http://www.youtube.com/embed/eSDN6mxEg3E 11
Nitrification
The nitrification process occurs chiefly in oxygen-rich environments, such as the top-most layer of soil. Here, two groups of microorganisms, Nitrosomas and Nitrobacter, convert ammonia to nitrite (NO2-), then nitrite to nitrate (NO3-), respectively.12 These autotrophic bacteria obtain all their energy from the oxidation of ammonia, while also making available nitrogen to nearby plants. Whereas ammonia is positively charged and is therefore able to stick to negatively charged soil particles, nitrates are negatively charged and are subject to erosion and leaching.13 Nonetheless, nitrates are more readily taken up by plants than is ammonia.
Decay (Ammonification)
Unlike nitrogen fixation and nitrification, through which atmospheric nitrogen is converted into usable forms of nitrogen, decay is the process by which “organic nitrogen compounds of dead organisms and waste material are returned to the soil.”14 These organic nitrogen compounds are usually found in the form of protein and urea, and are converted into inorganic forms of nitrogen, including ammonia and ammonium ions (NH4-). Thusly, similar to nitrogen fixation and nitrification, the process of decay converts unusable forms of nitrogen into inorganic nitrogen compounds that can be used by various organisms.
Denitrification
The last process of the nitrogen cycle is known as denitrification. Denitrification is considered to be the reverse of the nitrogen fixing processes. Anaerobic, or oxygen-averse, bacteria found deep in the soil or in aquatic sediments convert nitrates to gaseous nitrogen, returning the di-nitrogen molecule back into the atmosphere. However, the amount of nitrogen gas returned to the atmosphere through this process is relatively small.
So there you have it, from the atmosphere to the geosphere and from the geospehre to the biosphere, the nitrogen cycle is arguably one of the most important biogeochemical interactions. From its abundance in the atmosphere to its role as a fundamental building block of life, nitrogen must constantly be distributed throughout the different spheres of the Earth in order to maintain the greater circle of life.